Epigenetics, the Hologenome, and the comeback of Lamarckian evolution
- evobioblogs
- Apr 27, 2019
- 10 min read
The process of evolution is as complex as the machinery inside a clock: shaped by many different components which drive separate mechanisms that constantly influence, trigger and inhibit each other. Unlike a clock, however, the evolution of life occurs inconstantly and unpredictably, its course being redefined by external factors such as the environment and chance at all times, as discussed in the previous post.
The fundaments of current-day evolutionary biology – Charles Darwin’s theory of evolution by means of natural selection and Georg Mendel’s principles of genetic inheritance – were united under one mathematical framework named the Modern Synthesis in the first half of the 20th century. It was then, that evolution became defined as the heritable change in a population of organisms over time. In other words, only change that was embedded in an organism’s genetic material – its DNA – could contribute to its evolution.
As discussed previously, Darwin was not the first to conceive a mechanism for evolution. In the same year of Darwin’s birth, the French naturalist Jean-Baptiste Lamarck published the first Theory of Evolution in history. His theory was composed of two principal concepts: the tendency of life to become ever more complex; and the capacity of all life forms to adapt to their environment. Lamarck’s idea of adaptation obeyed two rules: firstly, he believed that the strength, size and capacity of an organism’s organs depended on the extent to which they were being used. In other words, if a part of an organism’s developing body would be put little in use, it would reduce in size, while it would become larger, stronger and more functional, if it was used continuously. Secondly, Lamarck believed that these acquired changes could be passed on to the next generation.
With this theory, Lamarck had found a way to explain much of the diversity that he could witness in nature. He famously used his theory to explain the evolution of the giraffe’s neck: as the animals reached for high-growing leaves, their necks would slowly stretch and become longer. The next generation of giraffes would then inherit this prolonged neck, since it allowed the animals to feed better, and by further stretching over many generations, the giraffes finally acquired the long necks that we see today.
Left: Jean Baptiste Lamarck. Right: comparison of Lamarck's theory of acquired inheritance (up) and Darwin's theory of natural selection (down): whereas under Lamarckism, the evolution of a giraffe's long neck is attributed to the heritable stretching of its short-necked ancestors to reach higher-growing leafs, Darwinism assumes a random variation in the neck length of the ancestral giraffes and an environment that led giraffes with a longer neck to feed better, survive more often and produce more offspring, while short-necked giraffes would die off and not contribute to the next generation.
Although Darwin himself, together with most evolutionary thinkers of his time, believed that Lamarck’s mechanism of use and disuse played an important role in the evolution of organisms, his ideas of acquired inheritance became obsolete in the 1880’s after being proven wrong by the German biologist August Weismann. In his Germ Plasm Theory, Weismann stated that there were two lines of cells in every multi-cellular organism: the somatic cells, which made up most of the body and organs and carried out all its functions, and the germ cells, which were present in the reproductive organs and were transmitted, together with the heritable information they contained, to the next generation. Because the germ cells were distinct from the rest of the body and could not be affected by whatever changes the body acquired during its life time, it seemed impossible under Weismann’s theory for acquired changes to be passed on to the next generation. Weismann famously demonstrated this (among other ways) by cutting off the tail of mice and observing that the young were never born lacking a tail nor even with a reduced tail. As Weismann’s theory of inheritance became the norm among biologists, Lamarck’s mechanism of evolution was discarded and became with time little more than an anecdote mocked and ridiculed by many.
In very recent years however, our scientific horizons have expanded so far from what could’ve been thought possible in 1880, that we’ve stumbled upon quite unexpected findings that might put Lamarckism back on the table. Of course, we now have a much better idea of the mechanisms of inheritance: we know that DNA is the molecule that bears inheritable information and that it is being passed on to the next generation through copying and recombining the parent strands. The DNA then codes for our traits and characteristics through discrete regions called genes, which are expressed in the body as proteins. Whatever changes occur in a population of organisms can only contribute to their evolution if it can be tracked down to a modification of the genetic code.
August Weismann and a diagram of the Germ Plasm Theory: the germ cell line is passed on to the next generation, while the somatic cell line makes out most of an organism's body and does not contribute to the offspring.
Nonetheless, it has become increasingly clear to geneticists, that not all evolution can be traced back to changes in just our DNA sequence. In recent years, thanks to the development of new technologies that enable us to study molecular mechanisms in the cell, scientists have reached a better understanding of the physical state of the genome and the role it plays in the expression of genes. The genome is namely not a loose string of A, C, T and G’s floating around unprotected inside a cell. Generally, the genome is located within a cell compartment called the nucleus (except in bacteria and archaea, which don’t have any cell compartments)[1]. There, the genome is folded up by a complex of proteins to fit it into the tiny nucleus, and to prevent it from being damaged. How the genome is folded up also determines which genes in the genome are being expressed as proteins and which are not. Typically, genomic regions which are very densely folded up (named heterochromatin) cannot be reached by the enzymes responsible for gene expression and remain inactive, while looser folding (in the euchromatin) enables them to approach the genes. This explains, for example, why cells in a body belonging to different tissues can look so different and have such disparate functions while sharing the identical genetic material: they differ in the genes that are active or not. Apart from genome folding, there are many other mechanisms that turn genes “on or off” without involving any changes in the underlying DNA sequence. Such phenomena have all been bundled under the term “epigenetic”, and since their discovery the field of epigenetics has only kept expanding and flowering[2].

Simplified cross-sectional image of an animal cell. The nucleus is visible in the middle, and contains the genetic information of the cell.
But what do these epigenetic changes have to do with inheritance? If changes in gene activity are not being caused by mutations in the DNA, it isn’t possible for these to be inherited and therefore, for these to contribute to a species’ evolutionary course, right? Well, under the classical laws of evolution and genetics, that is entirely correct. Nonetheless, although it was originally thought that differences in gene expression only led to the differentiation of cells within a body, and was not passed on the next generation, more and more studies have provided evidence that this type of non-genetic variation can indeed be passed on to the next generation[3]. These findings challenged the classical view of evolution as a change through time that is embedded in the DNA of organisms, as it implied that also changes outside of the sequence play a role in the evolution of life.
However, even more astonishing were the findings that showed that differences in gene expression between organisms due to epigenetic changes (for example, a slightly different way of folding up the genome) could be caused directly by external forces, such as the environment. In plants, for example, it was shown that exposure to drought could lead to increased tolerance after no more than 11 generations due to the occurrence of so-called “epimutations” – changes in the physical state of the genome which affect gene expression without changing the DNA sequence. Another study showed that plants descending from generations which had been exposed to herbivorous caterpillars kept showing the same signs of herbivore resistance induced by epimutations despite never having been exposed to herbivory, proving that epigenetic changes acquired in an organism’s life time could be passed on to the next generation.
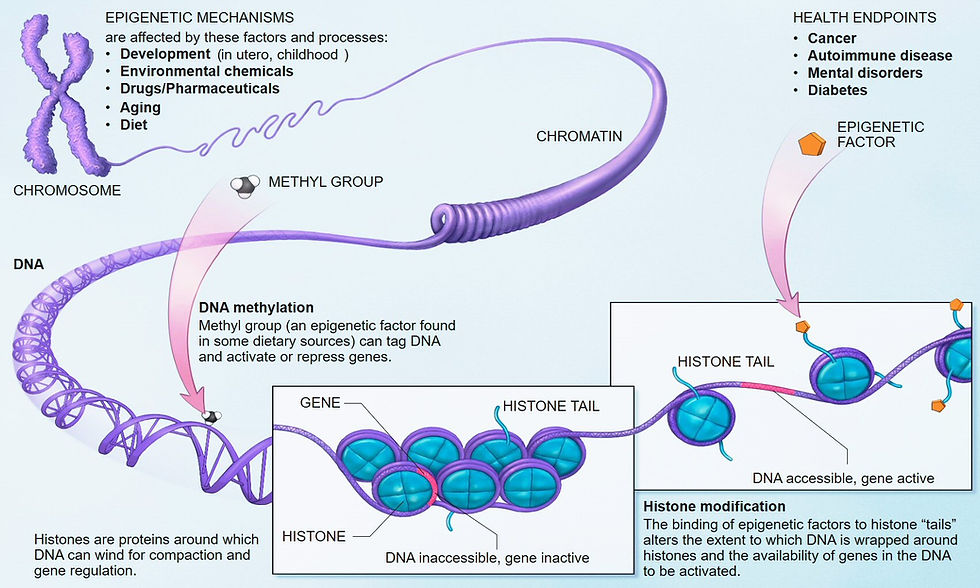
A summary on how the genome is folded up into chromosomes in the nucleus, and what epigenetic mechanisms affect the expression of genes (source: US National Institutes of Health).
Doesn’t all of this remind of Lamarck’s theory of acquired inheritance? In theory, if epigenetic changes caused by the environment affect an organism’s phenotype, they can also affect the organism’s fitness and thus be subject to natural selection. For that reason, epigenetic evolution has often been interpreted as Lamarckian. Nonetheless, this idea is far from accepted in the scientific community. It still remains very poorly understood how epigenetic changes are inherited and therefore what evolutionary role they can play. Most importantly, studies seldom provide evidence that epigenetic inheritance can last longer than a few generations, making it unfit to serve as a basis for persistent evolutionary change. Nonetheless, whether an epigenetic level of inheritance could bring back Lamarckism as a valid mechanism of evolution is still a topic of debate among scientist and will only be resolved once we have reached a better understanding of epigenetic mechanisms.
A second type of non-genetic inheritance that troubles the mind of geneticists has to do with the millions of micro-organisms that live inside and upon multicellular life forms such as animals and plants. Technically all animals and plants establish symbiotic relationships with micro-organisms. It’s becoming more and more clear that the community of micro-organisms in our gut is crucial not only for our digestion, but also for our health, our immune system, and even our mood. Plants are also very dependent on micro-organisms, which provide them with soil nutrients and forms of nitrogen that they themselves cannot otherwise obtain. The seemingly universal symbiosis between these microbial communities – named microbiota - and multicellular hosts has brought scientists to consider evolution on a new scale. If these organisms are so dependent on each other that they cannot carry out all necessary bodily functions to survive by themselves, should we then not consider them as a singular evolving unit?
The Hologenome Theory of Evolution describes the whole of host and microbial genomes as a new unit of evolution, named the hologenome. This means that host and microbial genes evolve as part of one life form (the 'holobiont') and determine each other’s characteristics. Importantly, an organism’s microbiota can change throughout its life time due to changes in diet and other environmental factors, causing the communities to change in composition, to lose certain micro-organisms or gain new ones. Therefore, the Hologenome Theory also takes on a Lamarckian perspective, claiming that it can after all be reconciled with classical Darwinism. An important condition for this theory to hold, however, is whether microbiota can be passed on from one host generation to the next. Mammalian babies get in touch, and probably acquire, their mother’s microbiota when passing through the vaginal canal at birth. What is less clear, is whether other life forms, such as plants, inherit microbiota systematically or not.
For this and many other reasons, the Hologenome Theory is even more controversial than the neo-lamarckian theories arising from epigenetics. Most importantly, it hasn’t yet been pinned down what exactly the terms ‘holobiont’ and ‘hologenome’ are supposed to represent, nor what conditions must or must not be met for the theory to hold. Like all neo-lamarckian theories (of which I have certainly not exhausted the list), the Hologenome Theory is at the moment subject to an ongoing discussion.
Whether we can say that Lamarck’s beliefs have truly made a comeback or not is not yet clear, and will not be for as long as we don’t understand the new discoveries we stumble upon as we search to solve the puzzle of evolution. One thing is certain: for many, it would’ve been hard to believe that scientists would one day pick Lamarck’s writings back up, ponder about them and consider reinstating them as a valid mechanism of evolution. In the beginning of the 20th century, a thought like that would at most have been a silly dinner joke. But that truly is the wonder of science; there is no ending to the list of questions nature prompts us to ask, and it is impossible to know what unbelievable things we might find next. Certainly, tiny folds of molecular strings in our cells determining how we look and behave, or entire cities of microbes influencing how we feel, were things no one would’ve believed to be true a hundred years ago - it is more likely that they would’ve been considered as mad ideas coming from a day-dream fantasy. Therefore, it is best not to discard and ridicule theories of the past. After all, Lamarck was a pioneer of science - even in the event that his thoughts of acquired inheritance would eventually be proved invalid once and for all – and he set out a path and a stream of thought that brought many others to develop their own ideas, and the whole of humanity to better understand the world that surrounds us. In other words, there is never an ending to discovery, and things that once seemed false, might become true at the next finding.
Footnotes:
[1] The bulk of genetic material in eukaryotic cells – that is to say, cells with compartments which are typical for fungi, plants and animals – is present in the nucleus and therefore called nuclear DNA. Nonetheless, animals and plants typically have a small part of the genome in the mitochondria and chloroplasts respectively, which is a different compartment in the cell.
[2] Such mechanisms include DNA methylation and histone modification. Importantly, differences in the behaviour of proteins which regulate gene expression due to changes in their structure are not regarded as epigenetic, as the change is influenced directly by the DNA sequence.
[3] In fact, the most recent definition of an epigenetic trait assumes its heritability, meaning that non-heritable changes in gene expression are strictly not regarded as epigenetic. It is important to note, though, that the term “epigenetic” still means different things to different people and is therefore quite vaguely used. For this article, I decided to stick with the consensus definition, but note that it is a hotly debated and unresolved question, what can and what cannot be classified as epigenetic.
For further reading:
Scientific paper on stress-induced epimutations in rice that lead to an increased drought tolerance:
Zheng, X. et al. (2017). Transgenerational epimutations induced by multi-generation drought imposition mediate rice plant’s adaptation to drought condition. Scientific reports, 7, 39843.
Scientific paper that demonstrates the heritability of stress-induced epimutations due to herbivory in tomato and arabidopsis plants:
Rasmann, S. et al. (2012). Herbivory in the previous generation primes plants for enhanced insect resistance. Plant physiology, 158(2), 854-863.
A biography of Jean-Baptiste Lamarck:
Delange, Yves (2002). Jean-Baptiste Lamarck. Actes Sud.
A general introduction to the field of epigenetics and its recent history:
Carey, Nessa (2011). The Epigenetics Revolution. New York: Columbia University Press.
A widely acclaimed popular science book on microbes and the relationships they establish with us:
Yong, Ed (2016). I Contain Multitudes: The Microbes Within Us and a Grander View of Life. New York: Ecco.
Comments